1. INTRODUCTION
Since the late 1950s, a large number of spacecraft (satellites) have been launched to observe near-Earth space (geospace, or the ionosphere and the magnetosphere), interplanetary space, and other planets. In situ and remote-sensing observations have revealed their structures, properties, and dynamics. So far only a few spacecraft at most were being operated simultaneously in coordination, but nowadays more spacecraft than ever, including small spacecraft and nano-spacecraft, are being operated and planned.
The magnetosphere is the region of space around Earth that is dominated by Earth’s magnetic field. It shields Earth from solar and cosmic energetic particles to some extent and prevents the atmosphere from eroding by the solar wind. Interaction with the solar wind and the ionosphere, however, results in dynamic disturbances within the magnetosphere, such as geomagnetic storms, substorms, and generation of energetic electrons in the radiation belts. Thus, observing the magnetosphere is important for understanding of space weather/environment and its forecast.
Not only Earth but also other planets with intrinsic magnetic fields, Mercury, Jupiter, Saturn, Uranus, and Neptune, have the magnetosphere under different conditions. Various plasma processes similar to Earth occur. Understanding planetary magneto-spheres help us understand the universality of Earth’s and planetary magnetospheres and the specialty of Earth’s magnetosphere in depth.
This paper briefly introduces current and future spacecraft missions for in situ and remote-sensing observations of Earth’s and other planetary magnetospheres, mainly scientific spacecraft currently being operated, approved for launch, and proposed as of February 2023. A few of the missions were terminated while I was preparing this paper, but I keep the descriptions of them in the paper for reference. I hope this paper will be a handy guide to the current status and trend of magnetospheric missions.
While the references and websites for each mission introduced in this paper can be found in each subsection, comprehensive information on missions for Earth’s magneto-sphere can be found at the following websites:
-
National Aeronautics and Space Administration (NASA) Space Science Data Coordinated Archive: https://nssdc.gsfc.nasa.gov/space/
-
“Explorers Program” at NASA Goddard Space Flight Center: https://explorers.gsfc.nasa.gov
-
“Missions” at NASA Jet Propulsion Laboratory and California Institute of Technology: https://www.jpl.nasa.gov/missions
-
“Mission Posters: Sun” at NASA: https://science.nasa.gov/get-involved/toolkits/heliophysics-mission-posters
-
“The Portal for Users of ESA’s Science Directorate’s Missions” at European Space Agency (ESA) Cosmos: https://www.cosmos.esa.int
-
“Current and Future Missions” at ESA European Space Operations Centre: https://www.esa.int/Enabling_Support/Operations/Current_and_future_missions
-
“Earth Observation Missions” at ESA Earth Observation Portal (eoPortal): https://www.eoportal.org
-
“Observing Systems Capability Analysis and Review Tool Space-based Capabilities (OSCAR/Space)” at World Meteorological Organization: https://space.oscar.wmo.int/spacecapabilities
-
Nanosats Database: https://www.nanosats.eu
In this paper I introduce Geotail, Cluster, Time History of Events and Macroscale Interactions during Substorms / Acceleration, Reconnection, Turbulence, and Electro-dynamics of the Moon’s Interaction with the Sun (THEMIS / ARTEMIS), Magnetospheric Multiscale (MMS), Exploration of energization and Radiation in Geospace (ERG), Cusp Plasma Imaging Detector (CuPID), and EQUilibriUm Lunar-Earth point 6U Spacecraft (EQUULEUS) for very recently terminated or current missions for Earth’s magnetosphere, and Lunar Environment Heliospheric X-ray Imager (LEXI), Gateway, Solar wind Magnetosphere Ionosphere Link Explorer (SMILE), HelioSwarm, Solar-Terrestrial Observer for the Response of the Magnetosphere (STORM), Geostationary Transfer Orbit Satellite (GTOSat), GEOspace X-ray imager (GEO-X), Plasma Observatory, Magneto-spheric Constellation (MagCon), self-Adaptive Magnetic reconnection Explorer (AME), and COnstellation of Radiation BElt Survey (CORBES) for future missions being prepared for launch and observations or proposed. Some of these missions are included in NASA’s and ESA’s fleets of heliophysics missions shown in Figs. 1 and 2, respectively.
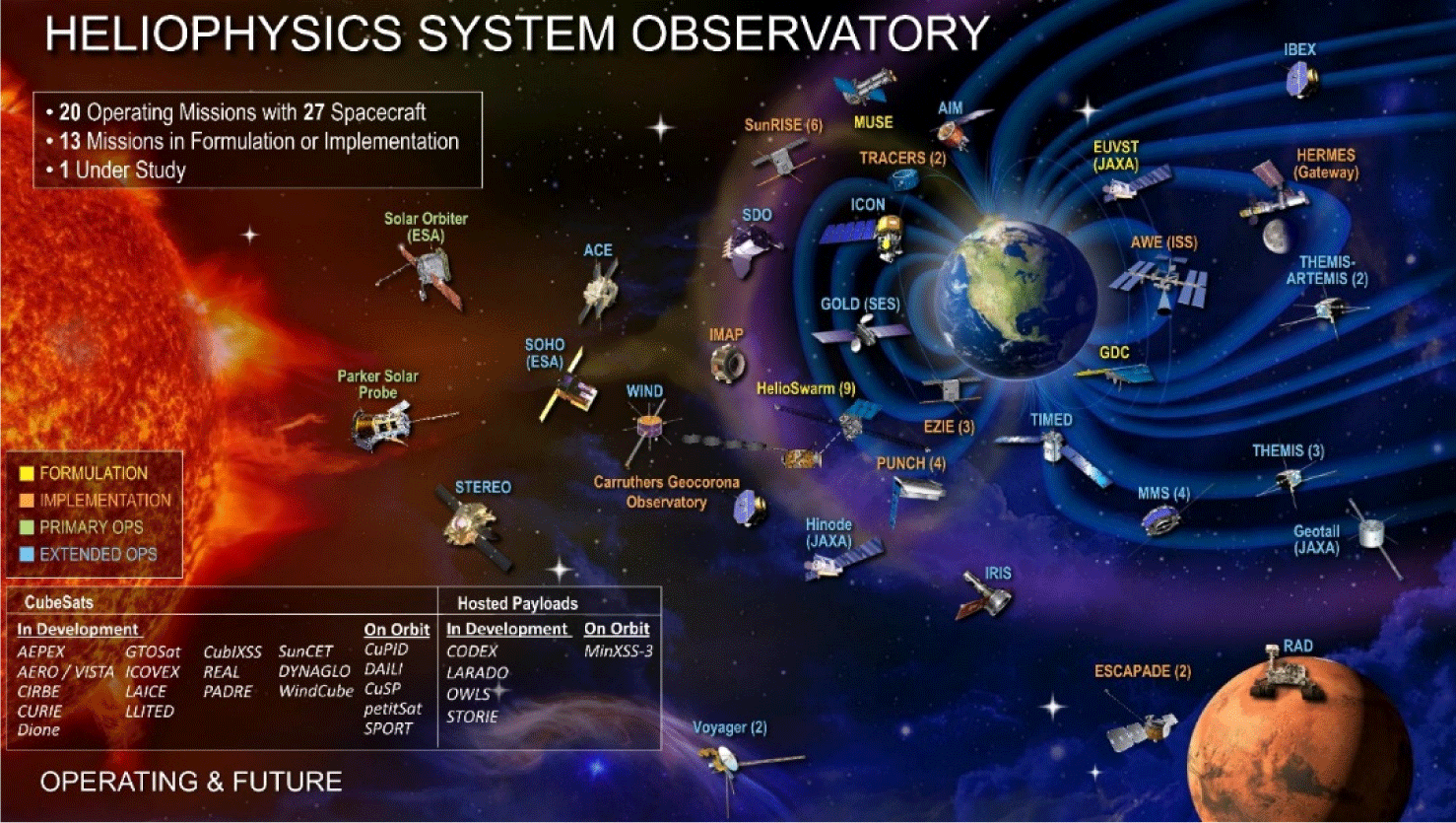
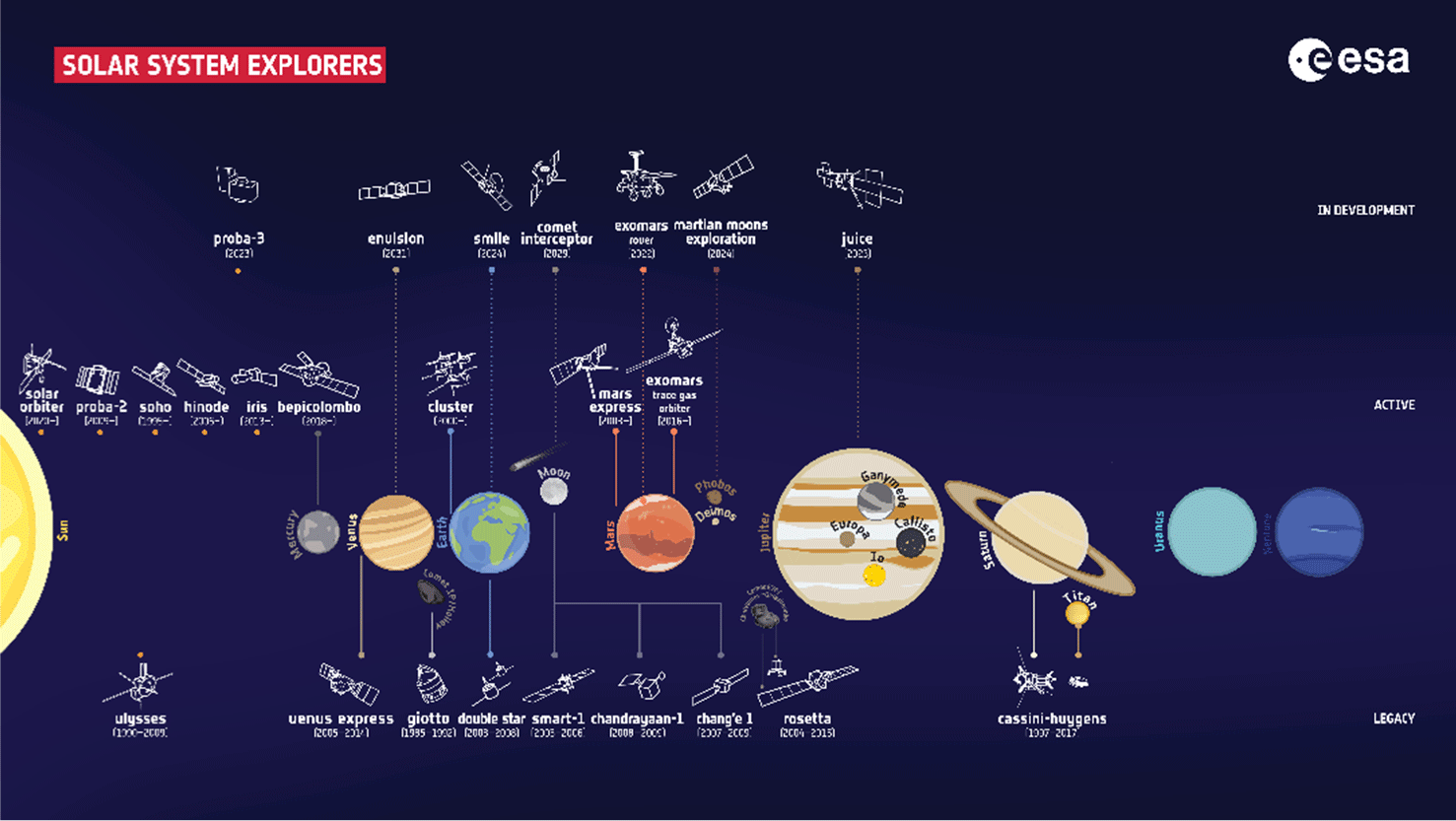
For missions for planetary magnetospheres, comprehensive information can be found at the following websites:
-
“Lunar and Planetary Science” at NASA Space Science Data Coordinated Archive: https://nssdc.gsfc.nasa.gov/planetary/
-
“Planetary Missions Program Office” at NASA: https://www.nasa.gov/planetarymissions/index.html
-
“Mission Posters: Solar System” at NASA: https://science.nasa.gov/get-involved/toolkits/planetary-mission-posters
-
“Current and Future Missions” at ESA European Space Operations Centre: https://www.esa.int/Enabling_Support/Operations/Current_and_future_missions
In this paper, I introduce BepiColombo for Mercury and Juno for Jupiter for current planetary magnetospheric missions; Jupiter Icy Moons Explorer (JUICE) and Europa Clipper for Jupiter, Uranus Orbiter and Probe (UOP) for Uranus, and Neptune Odyssey for Neptune for future missions. Some of these missions are included in NASA’s and ESA’s fleets of solar system missions shown in Figs. 3 and 2, respectively.
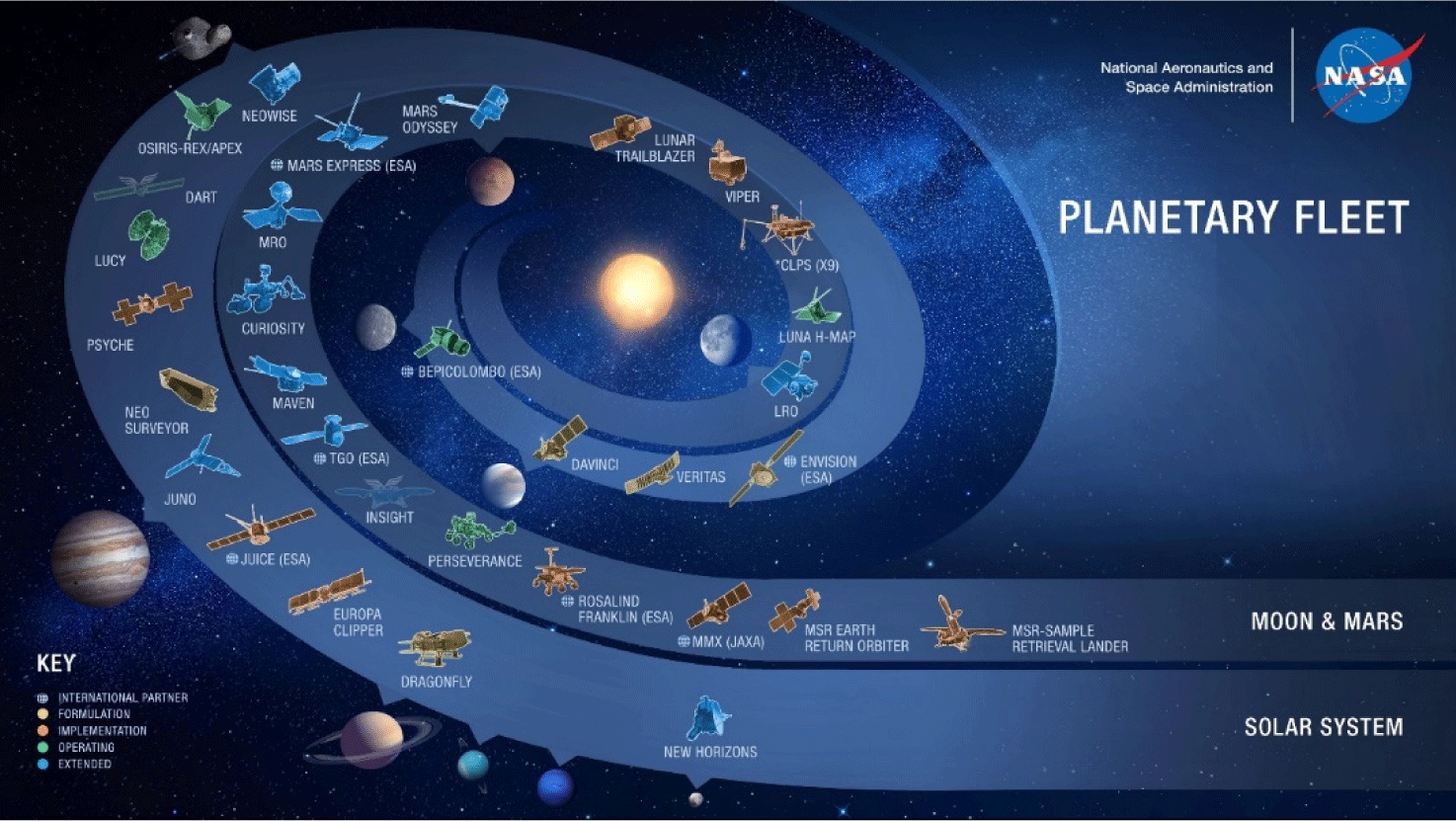
2. RECENTLY TERMINATED AND CURRENT MISSIONS FOR EARTH’S MAGNETOSPHERE
In this section, I introduce the recently terminated and current missions for Earth’s magnetosphere, Geotail, Cluster, THEMIS / ARTEMIS, MMS, ERG, CuPID, and EQUULEUS. Besides, the following magnetospheric missions had been operated since 2000, but I omit them here.
-
IMAGE (Imager for Magnetopause-to-Aurora Global Exploration; 2000–2005, NASA, USA, for imaging of the magnetosphere and aurora) [1,2]
-
Double Star Project (2003–2009, ESA and China, for observing the magnetotail and the inner magnetosphere by two spacecraft of Tan Ce 1 and 2) [3–6]
-
TWINS (Two Wide-angle Imaging Neutral-atom Spectrometers; 2006-2020, NASA Mission of Opportunity, USA, for stereoscopic imaging of the magnetosphere) [7–9]
-
Van Allen Probes (formerly Radiation Belt Storm Probes, RBSP; 2012-2019, NASA, USA, for the inner magnetosphere) [10,11]
-
DSX (Demonstration and Science Experiments; 2019–2021, Air Force Research Laboratory Space Vehicles Directorate, USA, for the radiation belt through very-low-frequency wave transmission) [12,13]
Furthermore, a lot of geosynchronous satellites are monitoring the inner mag-netosphere and the radiation belt, but I omit them as well: for example,
-
GOES (Geostationary Operational Environmental Satellites; National Oceanic and Atmospheric Administration (NOAA), USA) [14]
-
GK-2A (GEO-KOMPSAT-2A; 2018, Korea Meteorological Administration, Korea) [15]
Orbits of the current and past magnetospheric spacecraft are available at the Conjunction Event Finder http://ergsc.isee.nagoya-u.ac.jp/cef/orbit.cgi (Fig. 4) [16] and NASA Satellite Situation Center Web (SSCWeb) https://sscweb.gsfc.nasa.gov. Here note that in magnetospheric physics, distances in the magnetosphere and the surrounding space are often given in planets’ radii, instead of kilometer. For Earth’s magnetosphere (Earth’s radius RE is 6,371.2 km), the geosynchronous orbit is at 6.6 RE from Earth’s center; Moon’s orbit is at 60 RE; the subsolar magnetopause is typically at ~10 RE; the magnetotail magnetic reconnection sites are typically at ~20 and ~100 RE down the tail. For the instruments onboard each spacecraft mentioned below, typical energies of low- and high-energy particles are up to a few tens of keV and from tens or hundreds of keV to MeV, respectively. Typical frequencies of AC electric and magnetic fields of high-frequency waves are from tens of Hz to kHz or MHz. See the references of each mission for the figures, pictures, and more detailed descriptions of each spacecraft and instruments.
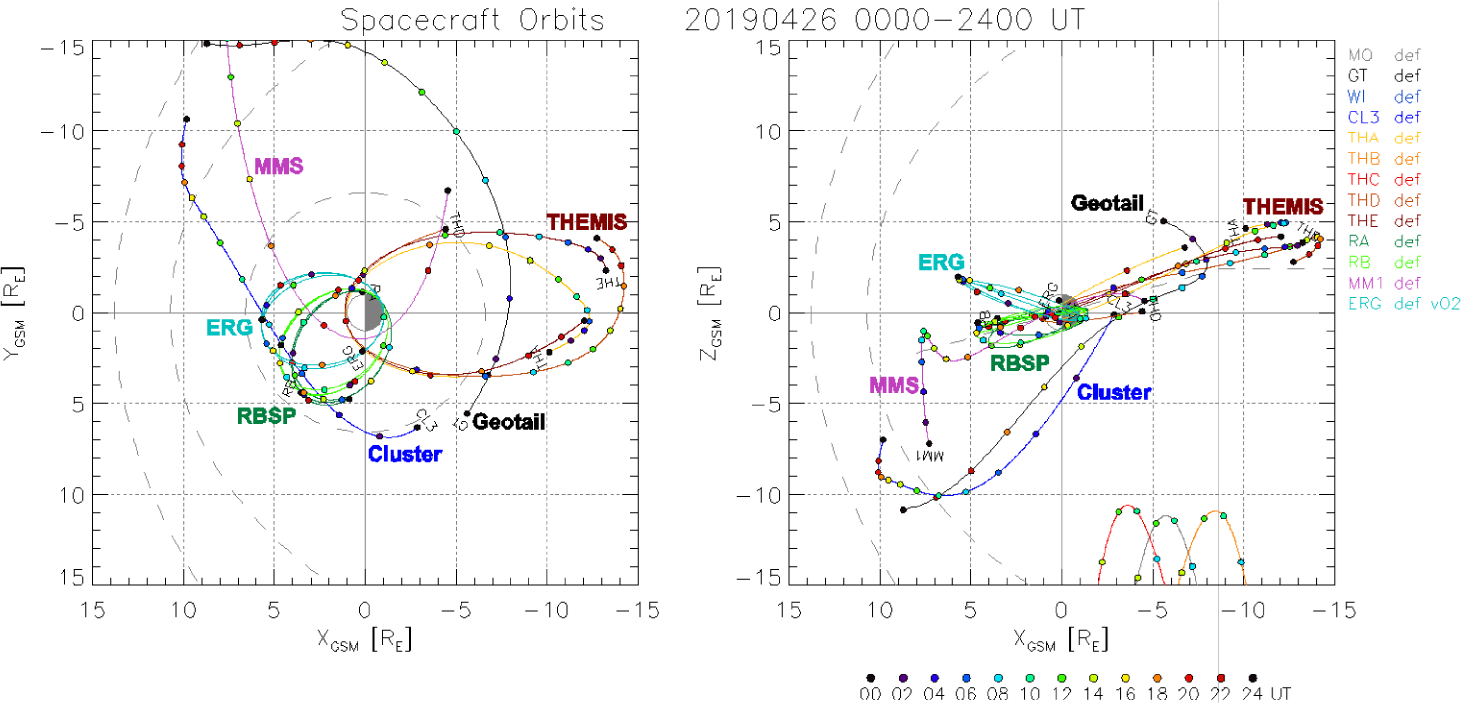
The Geotail mission [17–19] was a joint program of Institute of Space and Astronautical Science (ISAS) of Japan and NASA and participated in the International Solar-Terrestrial Physics (ISTP) Science Initiative, a cooperative scientific spacecraft project by NASA, ESA, and ISAS. The main target was magnetotail structure and dynamics, and Geotail was the first spacecraft that observed Earth’s magnetotail thoroughly. Geotail was launched on July 24, 1992, and its operation was terminated on November 28, 2022 after 30 years of observation. The orbit was 8 RE × 210 RE for the first 2 years to observe the distant magnetotail beyond the lunar orbit, and then the apogee went down to 32 RE to observe the near-Earth magnetotail in detail. The inclination was 29°. The spacecraft’s total wet mass (including propellant) was 1,009 kg at launch. Geotail had a comprehensive set of instruments for three-dimensional velocity distributions of low- and high-energy ions and electrons, and DC and AC magnetic and electric fields.
The Cluster mission [20–24] constitutes the Solar Terrestrial Science Programme (STSP), the first Cornerstone of ESA’s Horizon 2000 program, in collaboration with NASA and participated in ISTP. To study small-scale structures of the magnetosphere and its environment in three dimensions, the mission consists of four identical spacecraft that fly in a tetrahedral configuration. The original Cluster spacecraft were destroyed in the launch failure on June 4, 1996. As a recovery program called Cluster II, the spacecraft were successfully launched on July 16 and August 9, 2000 two by two. The orbit is 4 RE × 19 RE, and the inclination is 90°. The total wet mass of each spacecraft was 1,200 kg at launch. Each spacecraft has a comprehensive set of instruments for low- and high-energy ions and electrons, and DC and AC electric and magnetic fields. The observations at four tetrahedral vertices allow us to distinguish between spatial and temporal variations and derive differential quantities (gradients and rotations) in the observed system, such as the current density from ▽ × B. The separations of the four spacecraft are 600–20,000 km, so we can resolve ion kinetic scale.
The THEMIS mission [25,26] is the fifth NASA Medium-class Explorer (MIDEX). The mission consists of five identical spacecraft as well as ground-based auroral cameras and magnetometers installed in Canada and Alaska. The main targets are the triggering mechanisms of substorms and large-scale evolution of the magnetotail associated with substorms. The spacecraft were launched on February 17, 2007. The perigee is 1.1–1.5 RE, and the apogees are 10–12 RE for the three inner spacecraft and 20 and 32 RE for the two outer spacecraft. The five spacecraft were aligned along the magnetotail every four days in the winter of the Northern Hemisphere in 2008 and 2009. The inclination is 5°–9°. The total wet mass of each spacecraft was 126 kg. Each spacecraft has a comprehensive set of instruments for low- and high-energy ions and electrons, and DC and AC electric and magnetic fields.
In 2009, a spin-off of the THEMIS mission, ARTEMIS [27,28], started by repositioning the two outermost THEMIS spacecraft in lunar equatorial orbits at ∼55–65 RE, at a selenocentric distance of ∼1.1–12 lunar radii (100 km × 19,000 km in altitude) with an inclination of 10°. The separation of the two spacecraft are 500 km to 5 RE. This mission aims at the distant magnetotail, the solar wind, and the lunar space environment.
The MMS mission [29,30] is the fourth mission of the Solar Terrestrial Probes (STP) program of NASA’s Heliophysics Division. The main target is electron dynamics (kinetics) of magnetic reconnection at the dayside magnetopause and in the magnetotail, which is a fundamental process of plasma transport and energy conversion. Hence the mission consists of four identical spacecraft that fly in a tetrahedral configuration as the Cluster spacecraft, but the separations of the four MMS spacecraft are 10–400 km, much smaller than those of Cluster. The MMS spacecraft were launched on March 12, 2015. The apogee was 12 RE for the first two years to observe dayside magnetopause reconnection, and then it rose to 25 RE to observe magnetotail reconnection. The perigee is 1.2 RE. The inclination is 28°. The total wet mass was ∼1,250 kg. Each spacecraft has a com-prehensive set of instruments for low- and high-energy ions and electrons, and DC and AC electric and magnetic fields. In addition to distinguishing between spatial and temporal variations and deriving differential quantities, the MMS spacecraft can measure low-energy ions and electrons at 150 and 30 ms time resolutions, respectively, much higher than ever, to discuss ion and electron kinetics in the small magnetic reconnection site.
The ERG mission (nicknamed Arase) [31–33] is a small spacecraft project of Japan Aerospace Exploration Agency (JAXA). The main targets are the radiation belts and the ring current in the inner magnetosphere and space storms. The ERG spacecraft was launched on December 20, 2016. The orbit is 440 km × 5 RE in altitude. The inclination is 32°, higher than the Van Allen Probes, 10°. The spacecraft’s total wet mass was 350 kg. ERG has a comprehensive set of instruments for low- and high-energy ions and electrons, and DC and AC magnetic and electric fields. It also has a Software-type Wave Particle Interaction Analyzer (S-WPIA), which the Van Allen Probes did not have.
The CuPID mission [34,35] is a six-unit (6U) Cube spacecraft project of NASA’s CubeSat Launch Initiative (CSLI) [36]. To study macroscale properties of solar wind-magneto-sphere interaction, i.e., dayside magnetopause reconnection, the spacecraft measures soft X-rays emitted from the process of charge-exchange between solar wind ions and neutral atoms in the magnetosheath and the magnetospheric cusps. The spacecraft was launched on September 27, 2021, but it seems that communication between the spacecraft and the ground has not been established yet [37]. The altitude is 550 km, and the inclination is 98°. The total mass of the spacecraft is 8 kg. In addition to the soft X-ray imager, the spacecraft has energetic radiation detectors and a magnetometer.
The EQUULEUS mission [38–40], conducted by JAXA and The University of Tokyo, is a deep-space 6U spacecraft mission that demonstrates low-energy trajectory control technologies by cruising to the Earth-Moon libration point 2 (EML2). The spacecraft was launched as a secondary ride-on spacecraft on NASA’s Artemis 1 Space Launch System on November 16, 2022 [41,42] and will arrive at EML2 using lunar flybys and maneuvers one and a half years later. The total wet mass of the spacecraft was 11 kg. The spacecraft has a Helium ion (He+) imager for the plasmasphere named Plasmaspheric Helium ion Observation by Enhanced New Imager in eXtreme ultraviolet (PHOENIX) [43].
3. FUTURE MISSIONS FOR EARTH’S MAGNETOSPHERE
A lot of future missions for Earth’s magnetosphere are being prepared for launch and proposed, including not only large-class missions but also small spacecraft missions. Here I introduce LEXI, Gateway, SMILE, HelioSwarm, STORM, GTOSat, GEO-X, Plasma Observatory, MagCon, AME, and CORBES.
An X-ray imager similar to CuPID, LEXI [44], is being developed as part of NASA’s Artemis lunar program [41,42] and will be delivered and deployed on the lunar surface through Artemis’ Commercial Lunar Payload Services (CLPS) project in 2024. The instrument will take global soft X-ray images of the dayside magnetopause.
The Gateway [45], a part of NASA’s Artemis program [41,42], is an outpost orbiting the Moon to support landers and astronauts to the lunar surface and prepare for exploration beyond the Moon. The first Gateway modules are targeted to launch no earlier than November 2024. The Gateway will be inserted into a near-rectilinear halo orbit, i.e., highly eccentric polar lunar orbit at 0.5 RE × 11 RE [46]. A scientific instrument package, Heliophysics Environmental and Radiation Measurement Experiment Suite (HERMES) [47,48], will be placed on the Gateway. It measures low- and high-energy ions and electrons, and DC and AC magnetic fields to study the solar wind and the magnetotail.
The SMILE mission [49–53] is the second joint mission of ESA and Chinese Academy of Sciences (CAS). (The first ESA-CAS joint mission was the Double Star Project.) SMILE’s main targets are solar wind-magnetosphere interaction by soft X-ray imaging of the magnetopause and cusps, and resulting auroras, such as substorms, by ultraviolet imaging. The SMILE spacecraft will be launched in 2024 or 2025. The orbit is 2 RE × 20 RE, and the Inclination is 73° or 98°. The spacecraft’s total wet mass is < 2,250 kg. SMILE has a soft X-ray imager for the dayside magnetopause and cusps, an ultraviolet imager for global auroral distribution, and instruments for low-energy ions and DC magnetic fields.
The HelioSwarm mission [54,55] was one of the five Medium-Class Explorer (MIDEX-19) proposals for concept studies, supported by NASA Heliophysics Explorers’ program, and was selected as one of two proposals for launch. The mission consists of 9 spacecraft, 1 hub (mother spacecraft) and 8 nodes (small spacecraft), to study the complex three-dimensional structures of fundamental, universal plasma turbulence seen in the solar wind as well as the magnetosphere. The spacecraft are targeted to launch in 2028. The orbit is 17 RE × 60 RE with a low inclination. The mass of the hub and that of a node are 727 and 73 kg, respectively. The spacecraft measure low-energy ions and DC and AC magnetic fields at multiple scales ranging from MHD scale (3,000 km) to less than ion kinetic scale (50 km) simultaneously.
The STORM mission [56,57] was another MIDEX-19 proposal for concept studies, supported by NASA Heliophysics Explorers’ program, but was not selected for launch. The STORM mission proposed to study solar wind-magnetosphere interaction, and resulting auroral and ring current dynamics, based on global imagers. The spacecraft was planned to take a circular orbit at 30 RE, with an inclination of 90°. It was also planned to have a soft X-ray imager for the magnetopause and the cusps, a far ultraviolet imager for aurora, an energetic neutral atom imager for the ring current and the plasma sheet, and a Lyman alpha imager for the exosphere, as well as instruments for low-energy ions and electrons, and DC magnetic fields.
The GTOSat mission [58,59] is a 6U CubeSat mission in GTO, led out of NASA’s Goddard Space Flight Center through NASA’s Heliophysics Technology and Instrument Development Low Cost Access to Space (H-TIDeS LCAS) program in 2018. GTOSat will act as a pathfinder for future magnetospheric constellation missions and missions beyond low Earth orbit (LEO). GTOSat’s main target is radiation belt dynamics. The orbit is ∼185 km × 5.5 RE in altitude with a low inclination of < 25°. GTOSat measures high-energy ions and electrons and DC magnetic fields. The spacecraft was scheduled to be launched in August 2022, but it was not launched because it could not meet the 25-year orbital debris mitigation rule. The mission will look for another launcher [60].
The GEO-X [61,62] spacecraft, being developed by Tokyo Metropolitan University, JAXA, and several other Japanese universities, is a 50 kg-class, 12 U CubeSat for taking global images of the magnetopause and cusps through soft X-ray emissions. The spacecraft is proposed to be launched in 2023–2025 and then inserted into an elliptical orbit with an apogee of ∼40 RE and an inclination of ∼30°.
The Plasma Observatory mission [63] was proposed as a large-class mission of ESA Voyage 2050 science program [64] and was selected for further study for the M7 mission opportunity [65]. The mission aims to understand particle energization by magnetic reconnection, jets, waves and turbulence, shocks, and interplay between them in the magnetotail and at the magnetopause and the bow shock from simultaneous multiscale observations of electron and ion/fluid with nonlinearity and nonstationarity. For this purpose, at least 7 spacecraft form a constellation with two tetrahedrons sharing one corner. The mission considers two options for spacecraft: 7 identical spacecraft with a wet mass of ∼250 kg, or one mother spacecraft for high-resolution observations and 6 smaller identical daughter spacecraft for lower-resolution observations. The spacecraft are targeted to launch by 2037. The orbit is planned to be 8 RE × 25 RE with a low inclination. The spacecraft is planned to have improved instruments for comprehensive measurements of low- and high-energy ions and electrons, and DC and AC electric and magnetic fields.
The MagCon mission [66] was proposed to study mass and energy transport from the solar wind to the magnetosphere and energy storage and release processes in the magnetotail, i.e., substorms. A number of spacecraft are distributed between 6–8 and 25 RE with a low inclination. Originally this mission was planned to consist of 96 small spacecraft and reduce to 36 later. Because of huge cost, however, the current plan is to consist of 12 small spacecraft, which is the heritage from NASA’s past small spacecraft Space Technology 5 (ST-5) [67,68]. The total mass of each spacecraft is 35 kg. Each spacecraft measures low- and high-energy particles and DC magnetic fields.
The AME mission [69], another CubeSat constellation mission, was proposed for the Strategic Priority Research Program on Space Science II, CAS and is currently in the pre-phase A stage. The target is magnetic reconnection in Earth’s magnetopause and magnetotail. The mission consists of one mother spacecraft and 12 CubeSats to resolve cross-scale plasma processes from electron (1–10 km) and ion (100–1,000 km) kinetic scales to macro scale (1–3 RE). The orbit is 2.2 RE × 11 RE for the first 2 years for the dayside magnetopause and 2.2 RE × 23 RE for the next 2 years for the magnetotail, or 11 RE × 23 RE as another option. The inclination is 23.5°. The dry mass of the mother spacecraft and that of each CubeSat are 605 and 67 kg, respectively, and the total wet mass is 2,084 or 2,495 kg, depending on the orbit. The mother spacecraft and all CubeSats are equipped with a magnetometer and low-energy ion and electron detectors, which will be heritages from the SMILE mission. In addition, the mother spacecraft measures high-energy particles and AC magnetic and electric fields.
The CORBES program [70,71] was proposed by National Space Science Center (NSSC), CAS, coordinated by the Sub-Group on Radiation Belt (SGRB) of the Task Group on establishing a Constellation of Small Satellites (TGCSS) of COSPAR. Multiple institutes, universities, and industries are expected to contribute one or more spacecraft to form a constellation of ten or more CubeSats for very fast survey of the radiation belts. The orbit is near the equatorial plane with an apogee of ∼7 RE or similar to GTO. Each spacecraft is required to measure at least high-energy electrons and DC and AC magnetic fields.
4. CURRENT MISSIONS FOR PLANETARY MAGNETO-SPHERES
In this section, I introduce the current planetary magnetospheric missions, BepiColombo for Mercury and Juno for Jupiter. Until recently the following missions were operated, but I omit them here.
The BepiColombo mission [80–84] is a joint mission of ESA and JAXA for comprehensive exploration of Mercury and part of ESA’s Cosmic Vision program. It comprises two spacecraft: the Mercury Planetary Orbiter (MPO) led by ESA mainly for Mercury’s interior, surface, exosphere, and magnetosphere, and the Mercury Magnetospheric Orbiter (MMO, nicknamed Mio) led by JAXA for the exosphere and magnetosphere. The two spacecraft were launched on October 20, 2018, and are stacked and transferred by the Mercury Transfer Module (MTM). After the first and second Mercury flybys on October 1, 2021, and on June 23, 2022, respectively, and four more Mercury flybys, the two spacecraft will be released and injected into polar orbits in 2025. For MPO, the orbit is 480 km × 1,500 km in altitude. The total wet mass is 1,820 kg. MPO has instruments for low-energy ions, high-energy ions and electrons, and magnetic fields. For MMO, the orbit is 590 km × 11,600 km in altitude. The total mass is 275 kg. MMO has a comprehensive set of instruments for low- and high-energy ions and electrons, energetic neutral atoms, and DC and AC magnetic and electric fields. It also has a spectral imager of sodium, a major constituent of Mercury’s atmosphere. Combining the two spacecraft’s concurrent observations, we can study coupling of the solar wind, magnetosphere, exosphere, and surface. This is a vital difference between BepiColombo and MESSENGER.
The Juno mission [85–88] is the second mission in NASA’s New Frontiers Program for studying Jupiter’s origin, interior, atmosphere, magnetosphere, and aurora. The spacecraft was launched on August 5, 2011, and inserted into polar orbit around Jupiter in 2016. At the end of the mission, the spacecraft will impact Jupiter. The perijove and apojove are < 5,000 km in altitude on the dayside and 8 × 106 km (113 Jovian radii) on the nightside, respectively, and the inclination is 90°–105.5°. Hence Juno covers the nightside equator at 15–100 Jovian radii. The total wet mass was 3,625 kg. Juno has a comprehensive set of instruments for low- and high-energy ions and electrons, and DC and AC magnetic and electric fields, as well as ultraviolet and infrared spectral imagers for auroras.
5. FUTURE MISSIONS FOR PLANETARY MAGNETO-SPHERES
Here I introduce future planetary magnetospheric missions being prepared for launch, JUICE and Europa Clipper for Jupiter, and proposed missions, UOP for Uranus and Neptune Odyssey for Neptune.
The JUICE mission [89–93] is the first large-class mission in ESA’s Cosmic Vision 2015–2025 program. The mission studies Jupiter, including its magnetosphere, and the habitability of three of Jupiter’s largest moons, Ganymede, Callisto, and Europa, as well as Ganymede’s mini-magnetosphere. The spacecraft will be launched in 2023 and inserted into equatorial orbit around Jupiter in 2031. It will perform 35 flybys of Europa, Callisto, and Ganymede, raising the inclination. After that, it will orbit Ganymede. The total wet mass of the spacecraft is ∼2,900 kg. JUICE has a comprehensive set of instruments for low- and high-energy ions and electrons, and DC and AC magnetic and electric fields, as well as ultraviolet and visible-infrared spectrometers and an optical camera that can observe auroras.
The Europa Clipper mission [94–97] is under NASA’s Solar System Exploration Program. The mission studies mainly the habitability of Jupiter’s fourth largest moon, Europa, but can study Jupiter’s radiation and interaction of Europa’s atmosphere and ionosphere with Jupiter’s magnetosphere [98]. The spacecraft will be launched in 2024 and inserted into highly-elliptical orbit around Jupiter in 2030. It will perform ∼50 Europa flybys at altitudes of 25–2,700 km and also Ganymede and Callisto flybys. The total wet mass of the spacecraft is ∼6,000 kg. The instruments that can measure the magnetosphere include low-energy ion and electron detectors, radiation monitors, a magnetometer, and an ultraviolet spectrometer. Europa Clipper and JUICE will be able to collaborate in concurrent in situ observations of Jovian magnetosphere.
The UOP mission [99,100; see also 101] is proposed for the 2023–2032 Planetary Science and Astrobiology Decadal Survey [102] and endorsed as the highest-priority flagship mission. This mission aims to understand the ice giant planet Uranus comprehensively, including its rings, moons, and space environment. The spacecraft would be launched in 2031–2032 or 2032–2038 and start to orbit Uranus ∼13 or 15 years after launch. The spacecraft would take both polar and equatorial orbits by performing satellites flybys for ∼2–4 years. The total wet mass of the spacecraft and probe is 7,235 kg. The spacecraft has instruments for low- and high-energy ions and electrons, DC electric and magnetic fields, and high-frequency waves as well as an ultraviolet spectrometer for auroras. In addition, an atmospheric entry probe is deployed into Uranus’s atmosphere.
Neptune Odyssey [103,104] is a NASA Planetary Mission Concept Study (PMCS) for the 2023–2032 Planetary Science and Astrobiology Decadal Survey [102]. This mission aims to understand the ice giant planet Neptune comprehensively, including its rings, small moons, planet-sized moon Triton, and space environment. The spacecraft would be launched in 2033 and start to orbit Neptune in 2049. The altitude and inclination are altered using Triton flybys to cover a wide area. The total wet mass of the spacecraft is 3,816 kg. The spacecraft has instruments for low- and high-energy ions and electrons, DC magnetic field, and high-frequency waves as well as images of energetic neutral atoms and auroras. In addition, an atmospheric entry probe is deployed into Neptune’s atmosphere.
6. DISCUSSION AND SUMMARY
More spacecraft than ever are operated nowadays, but we have only a few to about ten spacecraft at most in the vast magnetosphere simultaneously in coordination. Even so, in scientific research, observations by past and current spacecraft have established or is establishing the overall pictures of the structure of each region in the magnetosphere and the magnetotail (e.g., the magnetopause; the radiation belts, the ring current, and the plasmasphere in the inner magnetosphere; the plasma sheet and the lobe in the magnetotail) and the dynamics of geomagnetic disturbances (e.g., storms, substorms, magnetic reconnection, and radiation belts electron acceleration). Fur-thermore, although encounters with the key regions are limited, observations by formation-flying spacecraft have revealed the magnetospheric structure and dynamics in each of MHD scale (> ∼1,000 km by THEMIS and other conjunctions), ion scale (∼100–1,000 km by Cluster), and electron scale (∼10 km by MMS) to some extent. These pictures, however, are fragmentary, combinations of observations under different conditions at different times. The magnetosphere is vast, magnetospheric phenomena are global, and the processes are complicated, so we always have to consider whether spacecraft continuously observed the structure and phenomena of interest in the right place at the right time.
In the future scientific research, in addition to establishing the more detailed pictures of the magnetospheric structure and each phenomenon further by more observations, it is essential to understand cross-regional coupling, cross-energy coupling, and cross-scale coupling. Cross-regional coupling is interaction between the solar wind, the magnetosphere, and the ionosphere through field-aligned currents, electric fields, and plasma waves. Through these processes, mass, momentum, and energy are transported from the solar wind through the magnetosphere to the ionosphere. Cross-energy coupling is interaction between low- and high-energy particles through wave-particle interaction, which is thought to be important for, for example, radiation belts electron acceleration. Cross-scale coupling is interaction between electron, ion, and MHD scales, that is, interaction between micro-scale phenomena and macro-scale phenomena and structure, which is thought to be important for, for example, magnetic reconnection. Thus, different regions and plasma populations with different energies are coupled to cause dynamic disturbances. For understanding of the coupling processes, we need more coordinated simultaneous multipoint observations in both small and large scales in full energy and frequency ranges at a very high time resolution. Here it is greatly helpful to integrate not only in situ and remote-sensing observations by multiple spacecraft but also ground-based network observations (e.g., magnetometers, radars, and cameras), theories, and simulations. If our understanding deepens and many constrains are given, we may possibly predict geomagnetic disturbances much more accurately as an ultimate goal. A series of reviews on specific subjects and future issues in magnetospheric research can be found in a book [105].
For magnetospheric missions, most of past and current spacecraft are of large or medium class, carried out in international collaboration. Small spacecraft, including nano-spacecraft and CubeSats, tend to increase for future missions, particularly formation-flying small spacecraft (e.g., HelioSwarm). For onboard instruments, many missions adopt combining newly-developed instruments and heritages from past missions. A recent trend seems to be imaging (e.g., SMILE) [106 for a review of imagers]. If it is realized, combining imaging and in situ observations could enhance our under-standing of magnetospheric dynamics. Active experiments [107,108; and references therein] seem to be few after the DSX mission.
Combination and balance of large- and small-class missions are important [109]. The opportunities of large-class spacecraft are limited, but this class of spacecraft can make comprehensive observations of low- and high-energy particles, DC and AC electric and magnetic fields, and images and is required to cover a broad range of science objectives. On the other hand, for small spacecraft, onboard instruments are limited to only a few and hence so are the science objectives, but there are more opportunities of launches due to low cost. In addition, small spacecraft can be used for demonstrating the feasibility of new mission concepts, technologies, and unique orbits for larger missions [110,111].
Furthermore, multipoint measurements are important for deepening our under-standing of space physics and environment [110]. Small spacecraft have the potential to accomplish constellation missions. Here spacecraft and instruments may not necessarily need to be identical, as proposed by CORBES. Besides, a fractionated constellation of small spacecraft [111–113], each of which makes different observations and is built by a different agency, may have the potential to perform better than large missions.